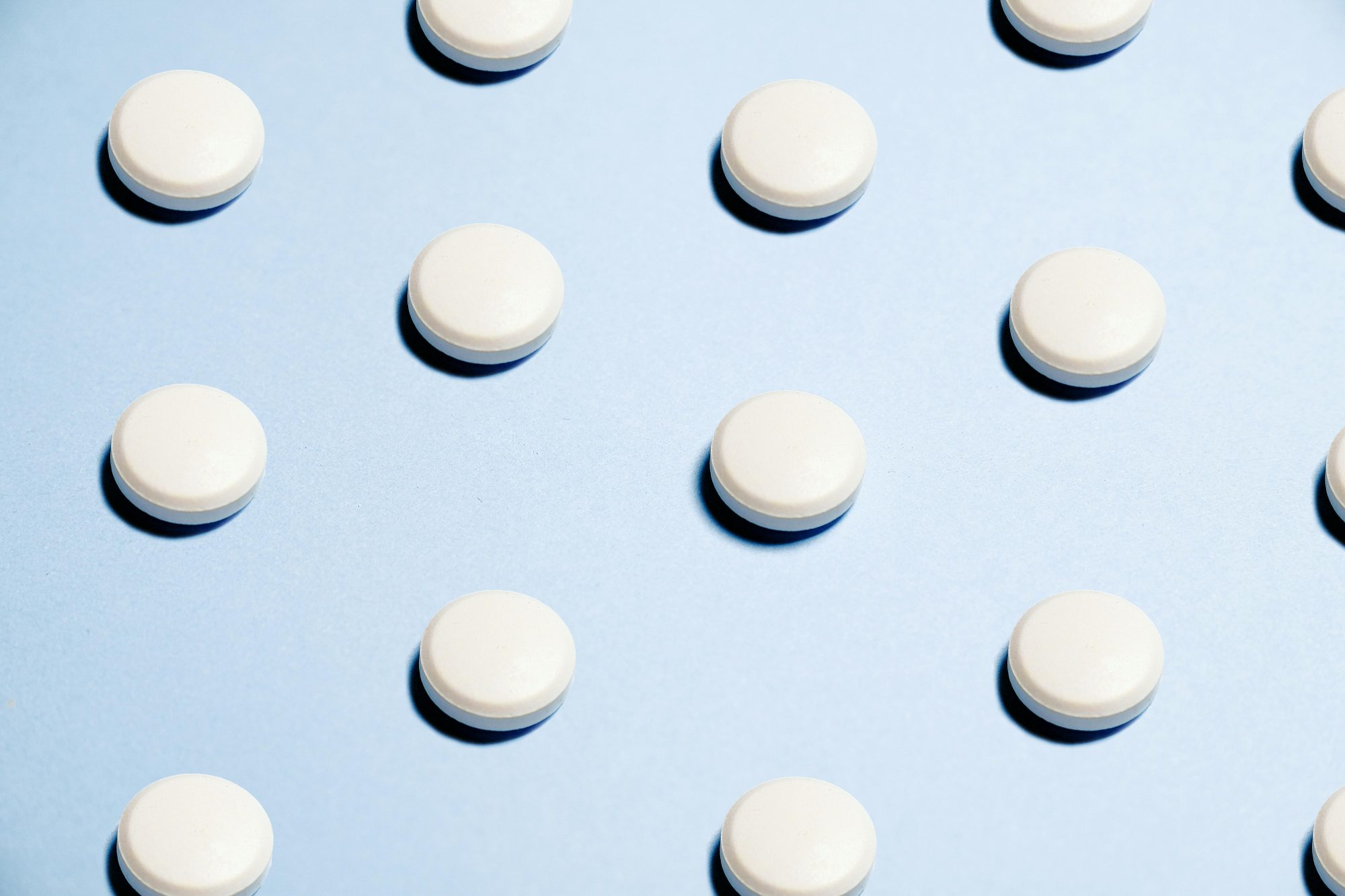
Read more
The following text is a transcript of the original podcast, available in French.
Our body is an incredible, very complex puzzle made up of 60 trillion pieces called cells. Cells assemble or join together to form all tissues and organs. The whole works in an organized, hierarchical and harmonized way, a bit like a symphony orchestra. Everyone produces their own sound, but the combination of all these sounds will define this music, which is called life. Thus, a cell will certainly work to preserve and make the organism function, but will also have to accomplish tasks for itself: producing its energy, producing its constituents, performing its function, possibly dividing, etc. To achieve all of this, a cell brings in an incredible number of factors … it’s a kind of miniaturized factory. And so, all of its functioning, enabled by our DNA, our chromosomes and whatever the organism … from bacteria to the most complex mammals like whales! or humans.
It’s a molecule that will take the form of chromosomes (we have 23 pairs) that carries our approximately 25,000 genes. This DNA or these chromosomes are found in the nucleus of each of our cells (with the exception of red blood cells). So the amount of DNA in our body is just gigantic: that is to say 2 meters in each cell … which we multiply by 60,000 billion and we arrive at a result which represents in all, approximately, 300,000 times the Earth-Moon distance.
DNA has a fairly passive role. In fact, it is the carrier of genetic information. It’s a bit like a barcode. When we are in a store and we choose an item, there is a barcode allowing the store to identify the product. The code here is generated by alternating thick and black bands. The person at the checkout therefore scans the barcode and has the information corresponding to the product. Let’s take the example of a state-of-the-art cell phone here. So we have: a code (bar), a reading system and a product.
The same goes for the cell. Except that the code, worn by DNA, is not a sticker with black bands. In fact, DNA is a long filament on which four different molecules will alternate … like a giant pearl necklace made up of 4 different colored pearls. These molecules or pearls, which are called DNA bases, are: Adenine (A), Thymine (T), Guanine (G), Cytosine (C). These molecules will alternate and succeed each other in a defined order. The code is based on a reading three bases by three bases. That is to say, the cell has a recognition system and goes to the basics of DNA in triplets. And so each triplet will correspond to information, a product and therefore in this case an amino acid. However, we have seen that DNA is very long and therefore by reading a gene in its entirety, we will end up decoding the information for a whole sequence of amino acids, which the cell will assemble. And ultimately, it forms a protein. A protein which can have various and varied roles in the cell: manufacture of its energy, manufacture of its constituents (membrane, nucleus, enzymes, etc.) but also proteins which will allow it to perform its function in the organism: proteins linking cells to ultimately create the skin, proteins to communicate a nervous message (neuron), proteins to achieve muscle contraction, antibodies when we are attacked, in short, full of things.
So here, like the store, we have DNA and its genetic code, a reading system and the product, namely the protein.
Before going any further, I would like to come back to one notion, it is the gene.
Of course the gene contains the code whose reading by the cell will lead to a protein. But that’s not all. Indeed, all cells obviously do not need to read all genes at the same time, some will be but others will not. So we have different categories of genes. On the one hand, there are important genes, necessary for life, and without them, we could never have created an embryo. And on the other, important genes at a given time or in a certain type of cell. Indeed, if we take the example of antibodies, they are produced only in immune cells and not in others. And this is also true for the proteins of muscle contraction, those that allow the production of hormones. All that to say that a gene cannot be read spontaneously like that. It will indeed only be read or expressed if necessary. A bit like when you’re in a store, you’re not going to take it all… it would take too many resources.
In fact, on genes, before the part containing the code is a part which will allow recognition of the gene and which is called promoter. This part is recognized precisely by factors that will be produced specifically. The goal is always harmony.
Now what if there is a problem with the genetic information?
To understand, let’s take our example of the store, its phones and its bar codes.
You can find your item and when you pay, there is a problem because the reading is impossible or does not lead to the right product because a bar has been slightly modified. So instead of ending up with your latest smartphone, you end up with a rotary phone. It’s annoying.
The same can happen for the cell, but the consequences can be far more serious than a simple disappointment linked to a telephone error. In fact, if a DNA base changes, it therefore changes the code and can modify the protein to varying degrees:
– First case, the change, and therefore what is called a DNA mutation, does not change anything. That is to say that despite the mutation we find the right amino acid or another that is of the same nature. So the protein is still produced and will be perfectly functional
– The second case, The mutation is problematic because the amino acid inserted is very different and therefore seriously modifies the protein, either that outright prevents the protein from being produced, and it will therefore be absent. Also, some proteins that are too modified, are not functional and in addition can take a very strange form. In the case of amyotrophic lateral sclerosis or charcot disease, the conformation of the mutated protein causes it to aggregate in the cell and its protein packets become fatal.
And so in all these cases of serious changes, that’s where genetic diseases come from.
80% of genetic diseases are fortunately rare and are therefore listed among rare diseases, precisely. These diseases are classified as such because they simply affect less than 1 in 2,000 people.
There are approximately 6,000 genetic diseases worldwide. Their causes are as diverse as the symptoms that result from it.
There is no classification of these diseases. But, I tried to prioritize things a bit and try to shed light on these pathologies. In fact, gravity depends on the mutation (s) and on which types of genes it is located. Let’s get rid of the absolutely primordial genes right away, since if they have a mutation, well life cannot be made. If a fertilized egg cell contains these kinds of genetic abnormalities, the embryo will not develop. This is unfortunately one of the main reasons for miscarriages (around 15% of pregnancies). And suddenly I suggest you look at two very distinct types of cases:
When the mutated gene is involved in the development of the embryo. And more particularly during the formation of one or more organs or different tissues. Therefore, the lack of this gene during these processes will affect the formation of an x or y organ and will be little or not functional from birth. I can give you the example of spinal muscular atrophy. Which is a disease linked to the mutation of a gene important for the development and growth of motor neurons. It’s already very precise. These motor neurons are supposed to stimulate the skeletal muscles and allow their contraction. Thus, children with the disease will have very great motor difficulties and their muscles will gradually waste away. In the most severe forms, patients end up paralyzed and die from respiratory failure.
The other category of genetic disease will involve a mutation in genes with a particular function in mature cells. I can give you the example of a disease that affects the immune system and is called DICS (Severe Combined Immune Deficiency). DICS is a very rare disease: the most frequent form (X-linked DICS) affects only one birth in approximately 200,000 each year. The most characteristic symptom is the excessive number of infections, due to malfunctions of the immune system, and this from the first months of life. In fact, patients are unable to produce a specific immune response that will result in the production of antibodies.
In this category, we can also cite the example of Duchenne muscular dystrophy. This disease affects 150 to 200 newborn boys in France every year (99.9% are boys). About 2,500 people are affected by the disease in France.
The disease rarely manifests before the age of 3. The boy begins to fall and has difficulty getting up. Muscle weakness gradually spreads to the lower limbs (pelvis and upper thigh), causing difficulty in running and climbing stairs, as well as frequent falls. Then the disease progresses to the back muscles and the upper limbs (shoulder blade and shoulder), making it difficult to grab objects or raise the arms. The respiratory muscles are also affected, as is the heart muscle, which contracts less effectively. This involvement begins with manifested by abnormal shortness of breath and palpitations. It is one of the major complications of the disease, leading to heart failure.
This disease is due to a mutation in the gene coding for dystrophin, located on the X chromosome. This protein is essential for the contraction of muscle fiber. In patients with Duchenne Muscular Dystrophy, in the absence of dystrophin, the fibers that make up the skeletal muscles, smooth muscles and heart muscle are damaged with each contraction and eventually destroy themselves. Muscle stem cells try to regenerate injured muscle tissue, but this process is quickly overwhelmed and degeneration eventually prevails.
Before getting to the heart of the matter, these therapies would not have been possible today in France without the telethon. To understand this well, let’s do a little history, like Florian. The AFM was founded in France in 1958 by parents who decided to declare war on genetic diseases. This association has with the first years, collected the first funds to support the sick and launched the first research. At that time, you should know that these diseases were absolutely unknown. With the arrival of the telethon, this unique audiovisual event, the collection has more than amplified and advances in medicine and research have multiplied. The genes responsible for many diseases have been identified one behind the other, the mechanisms of certain diseases have been understood, and the human genome has been completely dissected. The battle remained, the mother of all causes: treatment.
Obviously, the researchers did not wait for all this research to begin the development of certain gene therapy or cell therapy protocols … very innovative treatments.
The question that immediately comes to mind is why do we need necessarily innovative therapies?
Many research projects have shown that classical pharmacology offers no beneficial solution to patients suffering from rare genetic diseases. If we go back to our previous episode, the problem of genetic diseases is necessarily twofold. On the one hand, the “sick” gene is necessarily present in all cells. We remember the number of 60,000 billion cells! it’s just gigantic! In addition, the absence or malformation of the protein from the “sick” gene causes impressive damage to certain tissues or organs, damage that cannot be prevented by conventional means. Thus, it became obvious that the reintroduction of the gene by gene therapy or the grafting of cells freshly produced by cell therapy would probably be the only approaches to bring real benefit to patients. These are very very innovative methods that were only an impossible dream not long ago.
Immediately, I suggest you get into the heart of the matter by talking about cell therapy.
The goal of this approach is to transplant cells to restore the function of a tissue or organ. In this way, we hope to treat the patient sustainably with a single injection of therapeutic cells, obtained from stem cells from the patient himself or from a donor.
Before explaining things in more detail, let’s go back to the principle of stem cells. We hear a lot about it, without really knowing what it is.
By definition, a stem cell has two major characteristics: that of self-renewing itself indefinitely (which is practical because they can be cultivated in the laboratory) and that of being able to give birth to several different cell types (after receiving a message, often from chemical nature, to initiate this transformation. Generally, the recipe to manufacture for example neurons or skin cells is generally very complex and requires a lot of development).
There are different kinds of stem cells, depending on the number of cell types they can generate. First, we have pluripotent stem cells. They can give all types of cells. They are either of embryonic or synthetic origin. In France, since the revision of the bioethics law of 2013, research on embryonic stem cells is authorized, but is strongly supervised. Authorized projects must meet several criteria relating to their relevance, to ethics, to the objective of bringing major medical progress, to the fact that they cannot be carried out using another type of cell. The law provides that authorized research may be carried out using supernumerary embryos conceived within the framework of medically assisted procreation no longer the subject of a parental plan, after informing and obtaining the written consent of the couple concerned. This consent must be confirmed after a three-month cooling-off period and may be revoked without reason.
Because this agreement is recent and does not have a large number of stem cells, it was necessary to invent another way of producing these cells to be able to one day dream of cell therapy. These are called induced pluripotent stem cells. These cells are designed by skin sampling, for example from a patient and then genetically reprogrammed in the laboratory. This discovery has also earned its inventor, Prof. Shinya Yamanaka, the Nobel Prize in Medicine in 2012. However, these genetically reprogrammed cells can have long-term defects (problems of functionality and possibilities of becoming tumor). That’s why the top of the top is still embryonic stem cells.
Finally, the other type of stem cells are multipotent stem cells. They are already much more advanced in terms of development, since they are only going to have the capacity to give certain types of cell. We have them in our body and they allow the natural regeneration of certain tissues or organs. The best known, I would say, are those of the bone marrow, which is called hematopoietic stem cells. These cells cannot give birth only to blood cells, namely white and red blood cells. This is why in case of leukemia we often ask for donations of bone marrow. In fact, doctors irradiate the patient’s immune system and then transplant hematopoietic stem cells from a donor, which arrive in the patient’s bone marrow, give new non-sick cells and allow the patient to heal.
Because simple in principle and in application, this method has been used since the 1980s. However, thanks to the progress made by different sectors of scientific research (molecular biology, cell biology, genetic engineering, or medicine). Many trials are emerging and offer real hope to many patients, who for the moment are without solution. However, one of the limits, and of size, is that at the moment, it is not possible to manufacture very large quantities of cells. However, this can be enough for several diseases, let me convince you.
On October 21, 2014, Professor Philippe Menasché and his team from the Cardiovascular Surgery Department of the European Georges Pompidou Hospital performed a heart cell transplant derived from human embryonic stem cells. This operation was performed on a 68-year-old woman with severe heart failure. Ten weeks after the operation, the patient was doing well and her condition had improved significantly, without any complications having been observed. This promising advance has since been refined and extended to other patients.
The AFM Telethon was also a pioneer on this issue of cell therapy. The Institute of Stem Cells for the Treatment and Study of Monogenic Diseases (I-Stem) was inaugurated on January 1, 2005. It is a research and development center, dedicated to the development of well-founded treatments on pluripotent stem cells and applicable to rare diseases of genetic origin. Among the themes developed in this institute, I will simply take the example of relative diseases of the retina. ISTEM scientists have developed a process for making pigmented cells from the retina, stem cells, the black cells that are seen through the pupil. Concretely, they placed these newly manufactured cells on a membrane which is then grafted under the retina.
This method has proven to be tremendously effective in model animals of retinitis pigmentosa. After 13 weeks, the vision of these animals had greatly improved. Today, this method is being tested on a clinical level, in collaboration with the Institute of Vision. This treatment could also help treat all genetic diseases affecting the retina as well as age-related macular degeneration, which affects more than 1.5 million people in France.
Cell therapy trials are increasingly being launched around the world. Hoping to soon be able to graft muscle fibers for patients suffering from Duchenne muscular dystrophy or neurons for people affected by Alzheimer’s or Parkinson’s disease. Progress is still to come, for sure, but already this technique offers good results.
The principle of gene therapy is quite simple to imagine. Indeed, since a “sick” gene causes a disease, to suppress the disease it is “simply” enough to replace it in all cells. So, say that it takes about 5 minutes, gene therapy has run into overly complex problems for years. On the one hand, I recall the number, 60 trillion! So impossible to consider injections one by one, as we could do in IVF with an egg!
In addition to this problem of incorporating drug DNA into as many cells as possible, DNA has physical properties which make the task even more complex. Indeed, being a long filament, DNA is a fragile molecule which can break easily. Furthermore, like any organism, we have a defense system against foreign genetic elements. Thus, the half-life of DNA in the cytoplasm of a cell (i.e. the internal part without its nucleus, i.e. the largest volume) is very short. All this explains how long it has taken to implement!
To better understand this long journey, you have to go back to the 1950s! At that time, scientists had already raised the idea of changing the genetic makeup of a patient’s cells to eradicate disease. However, the knowledge and techniques of the time made this mission impossible. Then, with the development of biotechnology in the following decades, it gradually became easier to manipulate DNA. Scientists were therefore able to better identify (first step), sequence well, understand better and modify genes better. However, the most complex step was to vectorize DNA, which is to put it with something to get it into as many cells as possible. So we had lots of techniques, tests… especially with gold beads on which the DNA was stuck then these nanobeads were propelled using a cannon on the target tissue (all at scale medical course, nobody talks about war material). Obviously, the results were promising in the sense that slight benefits were seen, but were far from what you would expect from gene therapy.
To treat patients, the scientists were looking for small particles that could contain DNA and were able to walk around the body to bring DNA into as many cells as possible. In the end, the solution was not so simple, but at the same time very obvious! We know them all. We rub them daily: they are viruses! In fact, a virus is a small particle, about 1,000 times smaller than a cell. Viruses are fairly simple in their design. It’s a solid envelope called a capsid that contains the genetic material of the virus, that’s it. Because of their small size, these agents are capable of diffusing well into the body and injecting their DNA into a host cell. This for the purpose of reproduction, since this DNA will be read by the cell, will produce the proteins of the capsid and will assemble new viruses. And suddenly, if you understood all of this, you understood gene therapy. Indeed, the principle here is to take a DNA “drug”, that is to say a gene in its native conformation and which we will insert into a viral capsid. This is not harmful to health because the viral genes have been deleted. What remains of the virus is only its envelope, the capsid. It looks a bit like the Trojan horse, it’s just an appearance. Thus, we no longer speak of viruses, but of gene therapy vector. And so it becomes a drug that can be administered to the patient.
But, it has taken time since the first tests in the 90s!
Viruses are a bit like humans, they come in all sizes. Initially, scientists worked with fairly large vectors, from adenoviruses. At the time of the first attempts at intramuscular injection of adenovirus containing the dystrophin gene in animal models of Duchenne muscular dystrophy were carried out. A good restoration of dystrophin was observed in the injected muscle, which made it possible to recover a little muscle strength. The vector, on the other hand, was not capable of diffusing into the organism, and therefore of restoring dystrophin in the other muscles. Which therefore limited its use.
In parallel with this work, the first gene therapy trials on cancer brought very conclusive first elements. Teacher. Steve Rosenberg is the pioneer. He has indeed, with other scientists of course, used vectors derived from retroviruses to mark lymphocytes in cancer patients and also restore a sick gene in a 4-year-old girl suffering from severe immune deficiency. These tests were successful and were the start of a great adventure.
In the 90s and 2000s, the teams of Pr. Alain Fischer and of the Italian and English teams developed a treatment by gene therapy, DICS, an immune system disease which I had told you about in the previous podcast. Children with this disease have an almost nonexistent immune system and must live in sterile environments, bubbles. Concretely, these teams took the patient’s bone marrow and then put the hematopoietic stem cells in contact with lentiviruses containing the native version of the gene whose mutation caused the disease. Then these cells transformed by the vector were then reimplanted. Day after day, the patient’s immune system was rebuilt and the children were able to come out of their bubble. Today this protocol has been exported worldwide and has been able to cure dozens of patients. This technique, in which cells are extracted for transformation and healing the patient, is close to cell therapy, but is called ex-vivo gene therapy.
To carry out gene therapy which could be called classical, the researchers had to identify the vector of viral origin which would offer the best diffusion in the organism. Shortly before 2010, the discovery of so-called AAV vectors made it possible to put an end to this quest. These non-pathogenic environmental viruses are very small and therefore spread well throughout the body. However, the direct consequence of this is that the genes that can be carried will have to be small, too.
These vectors are used in numerous clinical trials. Among them, I would like to cite the example of spinal muscular atrophy, which we discussed in the previous podcast. It is a disease that affects in particular the motor neurons, which are located in the spinal cord and stimulate the contraction of skeletal muscles. Researchers from the Institut de Myologie de Paris, Généthon and some American teams have used this AAV (type 9 to be precise) and we are introducing the gene, SMN here, in its native version. The preclinical results achieved on mice are just spectacular. The mice with the disease, which were treated, had a drastically increased life expectancy (from 14 days to more than 300-400 days) and restored motor activity. This treatment has now arrived on the market in the USA and should arrive soon in Europe. Also, this treatment, and this is also its strength, is that it is administered only once!
Finally, we will finish with Duchennes’ Myopathy, which we also discussed during the previous podcast. The problem with this disease is that the gene encoding dystrophin is too large, including to be integrated into an AAV. However, researchers have identified for several years, a shortened form of this protein and which is functional: microdystrophin. Its gene has therefore been integrated into an AAV and has already shown initial results that are more than promising. Very soon, a clinical trial bringing together 30 patients will be launched. However, this path is not the only one that is in the implementation phase. Indeed, because of its size always, it was necessary to imagine something else. But before arriving at this ultimate discovery, I have to make you an aside on genes. When the cell reads a gene to make a protein, there are steps that I kept secret. It’s not as direct as that! When a particular protein needs to be produced, the cell first performs a sort of photocopy of the gene. This photocopy looks a bit like DNA, except that its chemistry changes a bit, and it’s called RNA. This RNA is immature in the sense that there are a succession of parts which contain information corresponding to the protein and parts which do not contain information. These parts are respectively called exons and introns. It’s a bit like an electric Christmas garland you have LED bulbs (which look pretty) and between electric cables. And suddenly, when we want to make the tree prettier, we try to hide the cables and bring the LED bulbs together. For the cell it’s pretty much the same. In fact, it will get rid of the introns by cutting them and will collect the exons by gluing them together. The goal is to have continuous information to make the protein. Now if we go back to our dystrophin. In Duchenne’s disease, it has a mutation! And so the idea the researchers had was to make the cell believe that the piece of DNA, and therefore the exon, which has the mutation, is a part that is useless, and therefore intron. That way at the time of producing the final RNA, just before the protein is made, the mutation is removed, it is definitely missing a bit, but it is not very serious, and above all the protein becomes functional again. This technique is called exon jumping, has shown more than positive results and is now in the clinical phase.
In any case, you see, clearly gene therapy has a more than promising future. To date, a few thousand trials of gene therapy are underway, for a large number of diseases: oncology, cardiovascular diseases, genetic diseases (where the results are often the most spectacular) and the rest in other very varied indications such as infectiology (tetanus, AIDS), neurodegenerative diseases (Alzheimer, Parkinson, multiple sclerosis…) or even ophthalmology.
To meet this demand, AFM Telethon has developed Genethon Bioprod, a cutting-edge center for producing gene therapy vectors for humans. This center was inaugurated in 2013 and produces all the batches of vectors for all the gene therapy trials in progress.
https://genetique-medicale.fr/la-genetique-l-essentiel/les-notions-cles-de-la-genetique/article/les-notions-cles-de-la-genetique-medicale
https://www.science-et-vie.com/corps-et-sante/20-000-40-000-100-000-mais-combien-avons-nous-de-genes-46459
https://www.orpha.net/consor/cgi-bin/Education_AboutRareDiseases.php?lng=FR
https://www.santemagazine.fr/sante/grossesse-et-bebe/grossesse-et-sante/la-fausse-couche-ses-symptomes-ses-causes-son-deroulement-170756
https://www.inserm.fr/information-en-sante/dossiers-information/deficit-immunitaire-combine-severe-dics
https://www.inserm.fr/information-en-sante/dossiers-information/myopathie-de-duchenne
https://www.afm-telethon.fr/association/notre-histoire-632
https://www.afm-telethon.fr/guerir/biotherapies-935
https://www.inserm.fr/information-en-sante/dossiers-information/therapie-cellulaire
https://www.inserm.fr/information-en-sante/dossiers-information/cellules-souches-pluripotentes-induites-ips
https://presse.inserm.fr/therapie-cellulaire-de-linsuffisance-cardiaque-premiere-implantation-de-cellules-cardiaques-derivees-de-cellules-souches-embryonnaires-humaines/17502/
https://www.afm-telethon.fr/guerir/biotherapies-935
https://histoire.inserm.fr/de-l-inh-a-l-inserm/50-ans-de-l-inserm/les-grandes-avancees/la-therapie-genique-devient-enfin-une-realite
https://institut-servier.com/sites/default/files/publications/Hacein_FR.pdf
http://therapiegenique.free.fr/partie4/th-therareu.htm
https://www.afm-telethon.fr/actualites/victoire-2019-traitement-therapie-genique-pour-amyotrophie-spinale-139044
https://www.genethon.fr/produits/dystrophie-musculaire-de-duchenne/